Germany
September 2, 2024
Breed, Sow, Grow: Adventures and Challenges in Plant Breeding
In our previous blog episode "Need for Speed" we learned about the concept of selection gain as a measure of trait improvement in a population's average performance. To estimate the selection gain, breeders must understand the heritability of the trait, phenotypic variation, and selection intensity. Today, we will take a closer look at selection intensity and how precision breeding supports it. Let's dive into "Precision Play: The Art of Crop Makeover."
What is Selection Intensity?
Selection intensity refers to how strictly breeders choose the best individuals for breeding. When selection intensity is high, only the top performers are chosen, which can lead to greater improvement in the trait. When selection intensity is low, more average performers are included, leading to slower improvement.
Several key factors affect the decision to use low or high selection intensity:
- Accuracy and Reliability of the Phenotypic of Assessment: The more accurate and reliable the evaluation of traits, the better the results from high selection intensity.
- Heritability of Traits: High selection intensity works best for traits that are mostly determined by genetics, rather than the environment.
- Trait Stability: Consistent expression of traits across different environments makes selection more effective.
- Population Size: A large population is needed to maintain genetic diversity, which is essential for long-term breeding success. A broad genetic base ensures there are enough top individuals to choose from, even with high selection intensity.
Modern breeding technologies, like marker-assisted selection (MAS), genomic selection (GS), and gene editing, are especially useful when applying high selection intensities. More details on these technologies will be discussed later in this post.
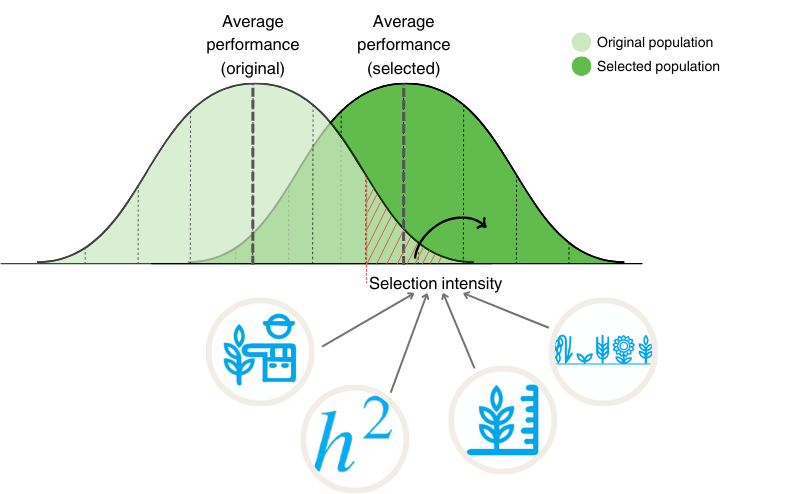
Image 1: The image shows how selection can improve the average performance of a group. The original group (light green) and the improved group (dark green), one generation later, are shown side by side. The x-axis represents performance, like yield, and the y-axis shows how many individuals are at each level of performance. A normal distribution is used to illustrate this shift. The selection intensity indicates how many of the top-performing individuals will be chosen for the next generation.
Consequences of Different Selection Intensities
High selection intensity, where only the top-performing individuals are chosen, offers clear benefits in plant breeding. It leads to faster genetic improvement, helping achieve breeding goals more quickly. However, this approach has its downsides. By focusing only on the best performers, genetic diversity can be reduced, which may limit the population's ability to adapt to new environmental challenges or to resist diseases. Additionally, a smaller breeding pool increases the risk of inbreeding, which can bring out harmful traits.
In contrast, low selection intensity, with more relaxed selection criteria, keeps a wider variety of individuals in the breeding process. This approach maintains genetic diversity, which is crucial for long-term adaptability and reducing the risk of inbreeding (in open-pollinating species). The trade-off is that progress in improving traits is slower, which can make it harder to meet breeding goals quickly, especially when there's an urgent need for agricultural solutions.
Therefore, finding the right balance between high and low selection intensity is key to achieving the best breeding results while safeguarding genetic diversity and ensuring sustainability.
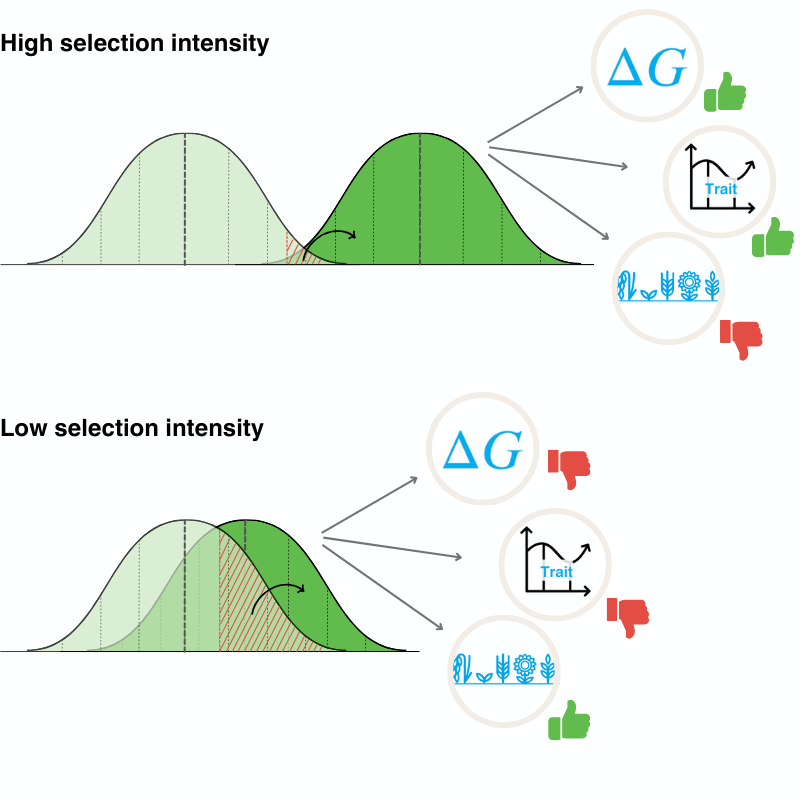
Image 2: Balancing selection intensity is vital for optimizing breeding outcomes and preserving genetic diversity. The image shows the difference between choosing only the top performers (high intensity) and including more average performers (low intensity). It highlights how each approach affects genetic gain (ΔG), trait stability, and genetic diversity.
Balancing Selection Intensity through Precision Breeding
Effective plant breeding programs often seek a balance between high and low selection intensity to optimize both immediate gains and long-term sustainability. One approach to this balance is precision breeding, a modern method that uses advanced genetic techniques to develop specific, desirable traits in crops more accurately and efficiently than traditional methods.
Precision breeding includes several groundbreaking techniques that are transforming plant breeding:
- Marker-Assisted Selection (MAS): This method speeds up the breeding process by identifying and selecting plants with specific genetic markers associated with desired traits, ensuring that the best individuals with the greatest genetic potential are chosen.
- Genomic Selection (GS): By using data from the entire genome, genomic selection predicts how well a plant will perform, allowing breeders to select the most promising candidates with greater accuracy and efficiency.
- Gene Editing: Tools like CRISPR-Cas9 allow for precise changes to a plant's DNA, making it possible to enhance traits such as yield, disease resistance, or drought tolerance by adding, removing, or modifying specific genes.
These techniques are revolutionizing plant breeding, making it possible to achieve better results more quickly while maintaining the genetic diversity needed for long-term sustainability.
Advantages of Precision Breeding
The benefits of precision breeding are numerous. Its targeted approach reduces the randomness and uncertainty involved in traditional breeding, making trait selection much more accurate. This method also dramatically shortens the time needed to develop new plant varieties with desired traits, speeding up agricultural innovation. Additionally, precision breeding allows for the efficient combination of multiple desirable traits into a single plant variety, resulting in superior crops.
Precision breeding directly impacts selection intensity by allowing for a more focused and efficient selection of plants with the best traits. This precision means breeders can more effectively identify and prioritize individuals with the highest genetic potential, increasing the overall selection intensity. By using genetic markers linked to specific traits, breeders can concentrate on key areas of the genome that influence those traits, making the selection process even more targeted and confident.
In terms of applications, precision breeding holds great promise for tackling various agricultural challenges. It can develop crop varieties resistant to pests, diseases, and environmental stresses, leading to more resilient agricultural systems. Precision breeding also offers the possibility of enhancing the nutritional content of crops, such as boosting vitamin levels or reducing allergens, which supports food security and improves human health. Additionally, by producing crops that require fewer inputs like water, fertilizers, and pesticides, precision breeding promotes sustainable farming practices, reducing environmental impact and ensuring long-term agricultural viability.
Interaction of Machine Learning and Precision Breeding
Machine learning complements precision breeding by using advanced algorithms to analyze huge amounts of genetic and trait data, making it easier to select the best candidates for breeding and creating high-performing crop varieties. Through genomic prediction, machine learning can accurately forecast how plants will perform based on their DNA, helping breeders quickly identify the right combinations of traits. These data-driven methods also uncover genetic markers linked to specific traits, boosting the accuracy of marker-assisted selection. By combining genetic, environmental, and historical data, machine learning refines breeding strategies, guiding crucial decisions such as which plants to cross and which traits to focus on.
The integration of precision breeding with machine learning marks a revolutionary shift in plant breeding. This powerful combination not only speeds up the development of better crops but also tackles major global challenges like food security, sustainability, and climate resilience. As we continue to push the boundaries of this technology, the partnership between precision breeding and machine learning promises to ensure the future of our global food systems—making them more resilient, sustainable, and ready to meet the demands of a growing world.
If you missed any of our previous episodes, read them here:
S1E1 - Breeding Brilliance: Unveiling the Crop Superheroes
S1E2 - Genius Genes: Unlocking Genetic Diversity
S1E3 - Phenomenal Phenotyping: The Science of Collecting Data
S1E4 - Genotyping Galore: Crafting Crops From Genetic Blueprints
S1E5 - Data Drama: Breeding's Behind-the-Scenes
S1E6 - Need for Speed: Accelerating Crop Breeding
Do you want to know how Computomics can support plant breeding for the future?
Check out our Climate-Smart Breeding page or contact us!